In order to study how metacommunity models can describe large, complex community networks, we have three main objectives.
- Test whether metacommunity mechanisms associated with mass effects, species sorting, and neutral models can be empirically detected and differentiated in a large-scale natural system.
- Compare metacommunity structure among aquatic macroinvertebrate, amphibian, and vascular plants.
- Assess how the importance of metacommunity mechanisms varies intra- and inter-annually
An ecological community consists of plants, animals, fungi, bacteria and other organisms interacting. Metacommunities are networks of communities connected to each other by organisms moving between different communities. Ephemeral ponds provide ideal sites for testing metacommunity models in scales relevant to conservation
The four metacommunities conceptual models:
Species Sorting Model:
This model assumes species dispersal is unlimited, and species persist in habitat patches with suitable environmental conditions and species interactions (Leibold et al. 2004, Holyoak et al. 2005). Therefore, we would expect relationships between community structure and habitat variables to be strongest in systems structured by species sorting mechanisms and the effects of landscape configuration to be small.
Mass Effect Model:
This model is similar to the species sorting model in that habitat patches differ in suitability for different species. However, certain patches serve as sources and others serve as sinks (lower quality habitat), leading to a weaker relationship between environmental conditions and the population sizes of some species (Holyoak et al. 2005).
Neutral Model:
This model assumes there is no species-environment relationship because there is no variation in species’ fitness between sites and there is limited movement restricted to random walks (Leibold et al. 2004). Under this model, we would expect wetlands that are geographically closer to one another to have more similar communities, with no relationship to environmental variables.
Patch Dynamic Model:
This model is related to the equilibrium theory of island biogeography and assumes that patches are environmentally homogeneous. Species colonization and extinction are functions of stochastic and deterministic processes. Since our wetlands are not environmentally homogeneous, this model is less applicable to our system and is not being tested.
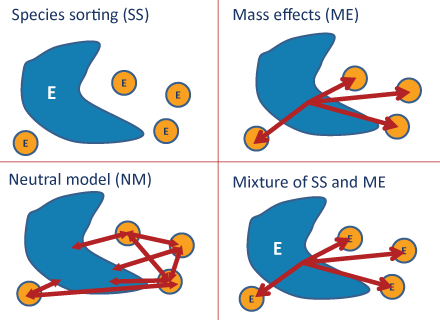
As a framework for understanding how networks of communities assemble and change, metacommunity theory helps resource managers make decisions about habitat and species conservation. Many natural areas persist as relatively undisturbed habitat patches within a larger altered landscape. Ecological communities in these patches fluctuate over time in response to local extinction, dispersal, environmental conditions and species interactions
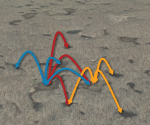
Four conceptual models attempt to explain metacommunity structure: patch dynamics, species sorting, mass effects, and the neutral model (Logue et al. 2011). These conceptual models are not mutually exclusive (Holyoak et al. 2005). The importance of different mechanisms may vary over time in response to environmental variability and disturbance.
Amphibian Sampling
- Surveys conducted at night identify frog species by their unique calls
- Minnow traps catch frogs, salamanders, and tadpoles
Macro-invertebrate Sampling
- Surface-associated Activity Traps (SAT) monitor macro-invertebrates that live on the water's surface
- D-nets (named for the shape of the ring on the net) sample the macro-invertebrates that live on the bottom of ponds
- Samples collected in the field are processed in the lab where macro-invertebrates are identified under dissection microscopes
Hydrologic Sampling
- Staff Gauges and ground water wells are installed in each wetland and used to record water levels
- Wetlands are surveyed for GPS coordinates and elevation
Water Quality
- Water temperature, pH, and dissolved oxygen measurements are taken in the field
- Concentrations of nitrogen, phosphorus, and chlorophyll are determined from samples brought back to the lab
Vegetation Sampling
- Wetland vegetation is sampled using transects and quadrats
Soils
- Wetland soil cores are extracted, sampled, and analyzed
The Chippewa Moraine Region is located east of New Auburn and north of Bloomer. Referred to as the “Blue Diamond” region due to the density of lakes, It is a forested landscape of steep hummocks, kettles, and ice-walled lake plains. Many of the kettles are large enough to hold small lakes, like Town Line or Shaft Lakes. Smaller kettles hold ephemeral ponds.
This region owes its abundant lakes to the last Ice Age. The glaciers left behind a thick blanket of sediment (moraine) over 200 feet (60 meters) thick in some places. The landscape of this moraine formed as large chunks of buried ice melted.
Glacial sediment contains a wide variety of particles from car-sized to microscopic and form low permeability soils. These sediments “filled in” any previous stream and surface water drainage network leaving a landscape able to retain surface water in lakes and ponds a long time.
The Ephemeral and Permanent Pond Locations
Each color in this map represents a different soil types in the Chippewa Moraine study area. The study ponds are outlined in red.
This map shows the area of surrounding land that drains into each wetland based on elevation data like that shown in the map below.
This map shows the elevation of the Chippewa Moraine Region study area. Higher elevations are white and red. Lower elevations are yellow and green. The research ponds are shown in blue.
Batzer, D. P., B. J. Palik, and R. Buech. 2004. Relationships between environmental characteristics and macroinvertebrate communities in seasonal woodland ponds of Minnesota. Journal of the North American Benthological Society 23:50-68.
Colburn, E. A. 2004. Vernal pools: Natural history and conservation. The McDonald and Woodward Publishing Company, Blackburg, VA.
Economo, E. P. 2011. Biodiversity conservation in metacommunity networks: Linking pattern and persistence. American Naturalist 177:167-180.
Holyoak, M., M. A. Leibold, N. Mouquet, R. D. Holt, and M. F. Hoopes. 2005. Metacommunities: A framework for large-scale community ecology. Pages 1-31 in M. Holyoak, M. A. Leibold, and R. D. Holt, editors. Metacommunities: Spatial dynamics and ecological communities, University of Chicago Press, Chicago, IL.
Leibold, M. A., M. Holyoak, N. Mouquet, P. Amarasekare, J. M. Chase, M. F. Hoopes, R. D. Holt, J. B. Shurin, R. Law, D. Tilman, M. Loreau, and A. Gonzalez. 2004. The metacommunity concept: a framework for multi-scale community ecology. Ecology Letters 7:601-613.
Logue, J. B., N. Mouquet, P. Hannes, H. Hillebrand, and The Metacommunity Working Group. 2011. Empirical approaches to metacommunities: A review and comparison with theory. Trends in Ecology and Evolution 26:482-491.
Naeem, S. 2001. Experimental validity and ecological scale as criteria for evaluating research programs. Pages 223-250 in R. H. Gardner, W. M. Kemp, V. S. Kennedy, and J. E. Peterson, editors. Scaling relations in experimental ecology, Columbia University Press, New York, NY.
Nicolet, P., J. Biggs, G. Fox, M. J. Hodson, C. Reynolds, M. Whitfield, and P. Williams. 2004. The wetland plant and macroinvertebrate assemblages of temporary ponds in England and Wales. Biological Conservation 120:261-278.
Peres-Neto, P. R., P. Legendre, S. Dray, and D. Borcard. 2006. Variation partitioning of species data matrices: Estimation and comparison of fractions. Ecology 87:2614-2625.
Schneider, D. W. and T. M. Frost. 1996. Habitat duration and community structure in temporary ponds. Journal of the North American Benthological Society 15:64-86.
Soininen, J., M. Kokocinski, S. Estlander, J. Kotanen, and J. Heino. 2007. Neutrality, niches, and determinants of plankton metacommunity structure across boreal wetland ponds. Ecoscience 14:146-154.
Werner, E. E., K. L. Yurewicz, D. K. Skelly, and R. A. Relyea. 2007. Turnover in an amphibian metacommunity: the role of local and regional factors. Oikos 116:1713-1725.